Radio Science investigates small changes in the frequency (phase), amplitude, and polarization of a radio carrier signal. Generally transmitted by a spacecraft, the signal propagates through interplanetary space and is eventually received on Earth. Changes in the signal's parameters arise from the relative motion between the spacecraft and ground station (Doppler effect), propagation through neutral (planetary atmospheres) and ionized media (ionospheres, solar wind, and solar corona), or reflection off planetary surfaces. These radio signals are typically used for communication between ground stations on Earth and the spacecraft, enabling the commanding of the spacecraft or the transmission of data from the spacecraft to Earth. The signal from the ground station to the spacecraft is usually employed for spacecraft control and is referred to as the 'uplink.' Conversely, the spacecraft transmits information and scientific data to the Earth on the 'downlink.'
Scientific areas of application
Radio Science can be utilized for various scientific purposes, including investigations of:
- Gravitational fields of planets, moons, asteroids and comets
- Neutral atmospheres
- Ionospheres
- Sun corona
- Surfaces (bistatic radar)
- Planetary rings
- Comet coma (dust and gas)
The frequencies used for these investigations are in the microwave range, with X-Band (8.4 GHz) and S-Band (2.3 GHz) commonly employed. More recent experiments also use Ka-Band (32 GHz). Ideally, two coherent frequencies (e.g., X- and S-Band) are used for the downlink, allowing for the separation of frequency disturbances proportional to the carrier frequency (classical Doppler effects) from plasma-induced effects (due to solar wind or ionospheric plasma).
Measurement Geometries
The geometry of the measurement depends on the scientific goals. For gravity field measurements , the high-gain antenna of the spacecraft points towards Earth, enabling the observation of small changes in the spacecraft's trajectory caused by the gravitational attraction of a celestial body (planet, moon, comet, or asteroid) (see Fig. 1).
Investigations of the neutral atmosphere and ionosphere occur in a so-called Earth occultation geometry. During these experiments, the spacecraft disappears from view on Earth behind the planet under study, allowing radio signals to propagate through the ionosphere and atmosphere of the planet (see Fig. 2). The same occurs when the spacecraft re-emerges on the other side of the planet. This leads to diffraction of the carrier signal, resulting in a frequency shift visible at the ground station. Using geometric optics or other more complex methods, the diffraction of the signal can be calculated, providing information about the refractive index of the atmosphere or ionosphere as a function of altitude. In the neutral atmosphere, this information can be used to calculate density, temperature, and neutral particle density, while in the ionosphere, an electron density profile is obtained. Radio occultations provide highly resolved profiles (resolution of a few hundred meters).
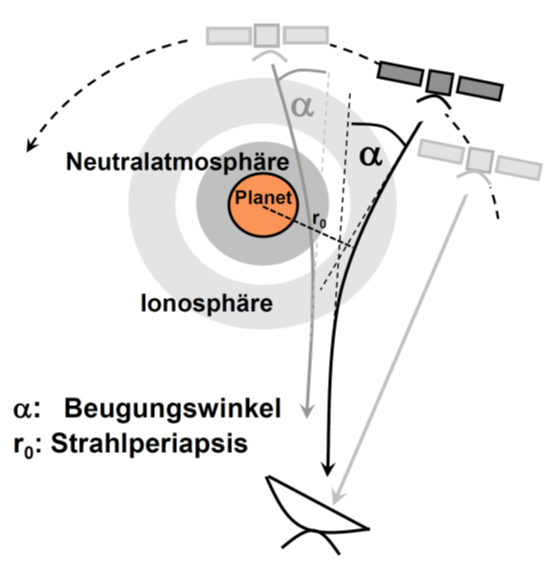
Figure 2: Measurement geometry in Earth occultations.
Bistatic radar measurements are used to determine surface properties. In this case, the high-gain antenna of the spacecraft points towards the surface of the planet. Signals are reflected and recorded on Earth using large ground stations (see Fig. 3). Under certain conditions, the dielectric properties of the surface can be determined.
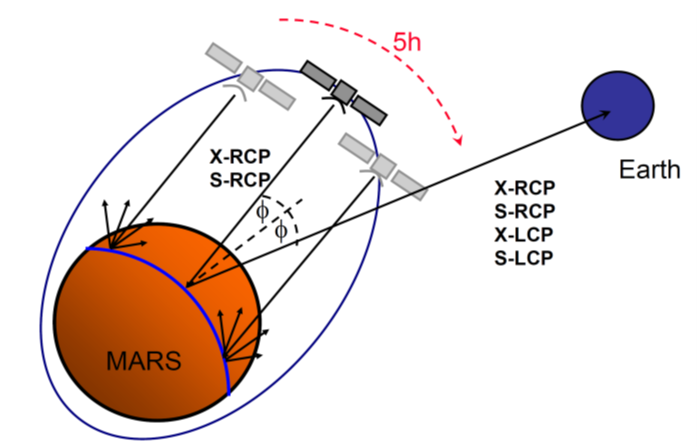
Figure 3: Measurement geometry for bistatic radar measurements. The high-gain antenna of the spacecraft is directed towards the surface, and signals reflected from the surface are recorded on Earth. This is done in two polarizations (right and left circularly polarized) and two frequencies (X- and S-band).
Whenever the angle between the spacecraft, the Sun, and Earth is small, and the spacecraft is farther from Earth than the Sun, the radio beam from the spacecraft propagates through the solar wind (see Fig. 4). This can be used to perform measurements of the solar corona . Two coherent frequencies are needed to separate plasma-induced effects from other disturbances.
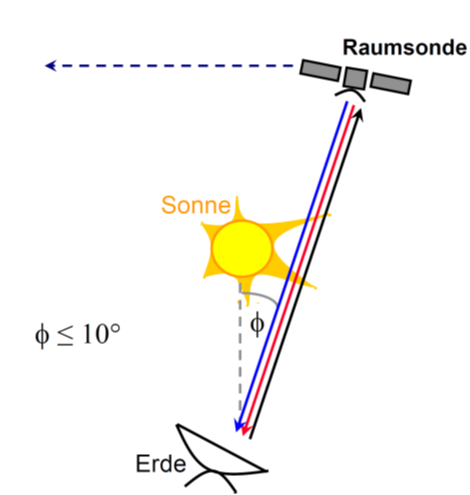
Figure 4: Measurement geometry for solar corona measurements. In this case, the spacecraft is in superior solar conjunction. This refers to measurements with an angle between spacecraft, Earth, and the Sun of less than 10°.
The Radio Link
For Radio Science experiments, a very stable radio signal is required. To generate the signal, a highly stable oscillator is necessary. If the spacecraft does not have such a stable oscillator, the stable signal is generated by a hydrogen maser in the ground station and sent via uplink to the spacecraft. There, the received signal is transformed into two coherent signals and instantly sent back to Earth. These measurements are referred to as 'two-way experiments' (see Fig. 5). This method is used, for example, in the Mars Express mission. A two-way experiment is also employed for solar corona measurements and gravity field measurements.
For occultation experiments and bistatic radar, a signal generated by the spacecraft itself is generally used. For this, the spacecraft must have an 'ultra-stable oscillator.' This allows for 'Oneway experiment', a method used, for instance, in the Venus Express mission.
Two-way radio link:
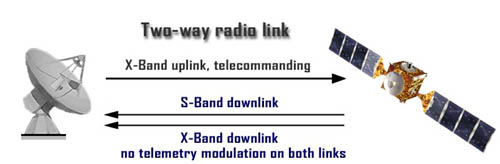
One-way radio link:
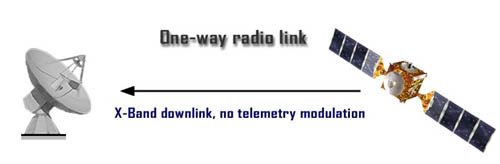
Figure 5: Different methods of the radio link.